Mitsuhiko Ikura
PhD, Hokkaido, Japan
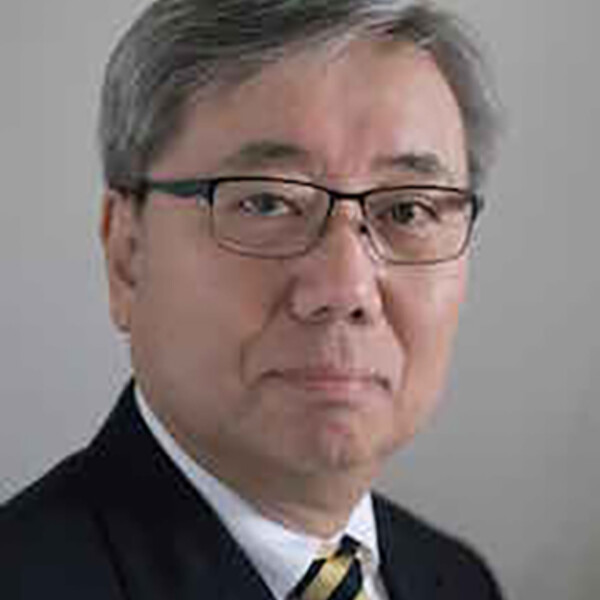
Research Synopsis
Cancer Cell Signaling and Structural Biology
Keywords: Pancreatic Cancer, Lung Cancer, Colorectal Cancer, Cancer Signal transduction, cell adhesion, cell polarity, gene transcription, cytoskeletal dynamics, calcium signalling, phospho-inositide signalling, RAS/MAPK signalling, mTOR signalling, NMR, X-ray crystallography, protein structure and function, live-cell fluorescence imaging.
We know that cancer is caused by genetic mutations and/or epigenetic alternations, and that different tumours exhibit significant diversities in genotype and phenotype. DNA sequencing technology and epigenetic regulation of genes revolutionized the way we think about cancer. However, it is clear that full understanding of cancer-associated genetic abnormities requires what happens to gene products, namely proteins, which are the major target of small-molecule and antibody therapeutic enterprise. In early 21st century, the concept of “personalized medicine” became a reality, with the development of molecularly-targeted small compounds such as EGFR and BRAF inhibitors. While these molecules showed high potency in treating specific subtypes of lung cancer and melanoma, respectively, they benefit only a small fraction of current cancer patients. Another challenge steams from cancer cell’s ability to quickly develop drug resistance. Identification and targeting of other signalling molecules and processes will lead to the next generation of selective cancer therapeutics. The future of molecularly-targeted therapy depends upon our greater understanding of proteins with specific molecular genetic abnormalities that drive different subsets of cancer.
In our laboratory, we are particularly interested in understanding how genetic alterations affect the 3D structures of proteins, and deregulate their associated signalling pathways. Our experimental tools include NMR spectroscopy, X-ray crystallography, cryo-EM, fluorescence resonance energy transfer (FRET) and total internal reflection fluorescence (TIRF) imaging microscopy. NMR, X-ray, and cryo-EM enable us to determine atomic-resolution 3D structures of key molecular players and analyze the dynamics and kinetics of their interactions, while in-cell FRET and TIRF microscopy let us visualize where and how the signalling molecules work in the cell in response to a stimulus. We combine structural and biochemical analyses with functional studies on mammalian cell lines and model organisms using advanced technologies such as gene knock-out and RNAi-based gene silencing. Presently our research focuses on signalling pathways involved in three key aspects of cancer: i) small GTPase signalling, ii) cell adhesion and polarity, and iii) calcium homeostasis. We believe that our molecular studies of cancer cell signalling will contribute to a deeper understanding of this life-threatening human disease. Details of specific studies are summarized below:
1. Small GTPase Signalling
The small GTPase K-Ras is one of the most frequently mutated oncogene products, with activating mutations most prevalent in human pancreatic (95%), thyroid (55%), colorectal (35%), and lung (35%) carcinomas. We intend to identify and characterize druggable target molecules that play a critical role in the Ras signalling pathway in cancer cells that are “addicted” to K-Ras activating mutations (for instance, G12V). The Ras signalling pathway involves many proteins, some of which may offer targets for synthetic lethality with K-Ras mutations in cancer cells. Guanine nucleotide exchange factors (GEFs) and GTPase activating proteins (GAPs) are obvious candidates for therapeutic targets, as they directly alter the activity of small GTPase proteins and hence effector proteins downstream in the signalling pathway. By disrupting or inhibiting one of those signalling proteins, it may be possible to specifically kill the oncogene-addicted cells by virtue of “synthetic lethality”. We believe that molecular and structural studies of these key signalling molecules are extremely important to better understand how the signalling pathways in certain cancers are “wired” for cell survival, and how we could exploit cancer-specific molecular pathways to selectively kill tumour cells.
We have recently developed a new real-time NMR methodology to assay small GTPase nucleotide exchange and hydrolysis, as well as the catalysis of these reactions by GEFs and GAPs, respectively. This assay uses the natural ligands GTP and GDP and has been successfully applied to a number of small GTPases including Ras, RhoA, and Rheb. Rheb, a member of the Ras sub-family of small GTPases, functions as an activator of mammalian Target of Rapamycin (mTOR), which is implicated in cell growth, proliferation, and tumourgenesis. We have characterized a number of mutations in the tuberous sclerosis complex 2 (TSC2) protein, a Rheb-specific GAP implicated in the congenital genetic disorder TSC in humans. Using NMR spectroscopy and X-ray crystallography, we are currently investigating regulatory mechanisms of several small GTPases such as Rheb, Ras and RhoA, and addressing questions of how somatic mutations found in GTPases or their GAPs/GEFs deregulate the GTPase cycle, thereby affecting normal cell function and leading to tumourgenesis. Examples include the RhoGEFs, PDZRhoGEF, and Lfc/GEF-H1, as well as RasGAPs and RasGEFs. Our NMR-based GTPase assays could offer a highly specific and reliable tool to perform functional assays for biomarkers and drug development.
2. Cell Adhesion and Polarity
The main cause of mortality in cancer patients is secondary tumours, which are mediated by metastatic epithelial cancer cells. As a matter of fact, epithelial cancers constitute ~85% of all cancers including primary tumours. For these reasons, a detailed understanding of epithelial cells is crucial to combat cancer. Like many other cell types, epithelial cells have the ability to adhere to one another and form a specific multicellular structure (epithelium), which is fundamental to normal cell development and morphogenesis of human tissues. Cancer disrupts normal cell adhesion and polarity functions, permitting the spread of cancer cells from the primary cancer site to other locations in the body (metastasis). Cell adhesion and polarity are both coupled with intracellular signalling and cytoskeletal assembly. Together these cellular processes control the shape and motility of cells in a dynamic manner. The cell-cell adhesion molecule cadherin and its associated factors called catenins play critical roles in maintaining the stable cell-cell interaction. Loss of E-cadherin function has been implicated with tumourgenesis and metastatic potential of cancers. Not surprisingly, recent studies have revealed that the cadherin adhesion system is coupled with cell polarity proteins that co-localize at the apical side of the epithelium. Using X-ray crystallography and NMR spectroscopy, we plan to determine the structural basis of the cadherin-mediated cell-cell interaction, and are working to determine the mechanisms by which the cadherin function is regulated through interaction with intracellular proteins and cytoskeletal components. Both E-cadherin and p120-catenin are well-known targets for Src protein kinases. We are investigating how Src-dependent protein phosphorylation alters the structure and function of the cadherin-catenin complex. We are also studying the Par complex, which consists of a number of cell polarity proteins. Structural characterization of these protein-protein interactions would be crucial in understanding how cell polarity and cell adhesion phenomena are dynamically regulated. We hope to reveal novel insights to support the development of therapeutics to reduce or abolish the metastatic potential of cancer cells.
3. Calcium Signaling
Cancer-causing mutations disrupt the homeostasis of normal cells, and remodel it to promote survival of the cancerous cells. Among many factors that control cellular homeostasis, the calcium ion (Ca2+) is a vital second messenger in the cell, whose concentration is tightly regulated, such that it generates cellular signals in response to external stimuli. Utilizing Ca2+signal pathways, metastatic cancer cells develop an enhanced propensity to migrate, and effectively detach from the tumour, enter the circulation system, and re-establish secondary growth in distant organs. The Ca2+ signalling pathway responsible for cancer metastasis appears to involve Orai1, and stromal interaction molecule 1 (STIM1), which are downstream of the inositol 1,4,5-trisphosphate (IP3) receptor (IP3R), G-protein-coupled receptors and receptor tyrosine kinases. Recent studies have revealed that Ca2+ high/STIM2 low expression. These molecular alterations in cancer cells lead to remodeling of intracellular Ca2+ homeostasis, resulting in altered gene expression in the cell. Interestingly, a point mutation of Orai1 (R91W) was identified in patients with the severe combined immune deficiency syndrome (SCID) and this mutation was found to impair Ca2+-release-activated Ca2+ (CRAC) activity. In order to understand how the concentration of 2+ in the cell is regulated in both a spatial and a temporal manner, and how this affects gene expression in cancer cells, we need to better understand the roles and precise regulatory mechanisms of various 2+ transporters (i.e., Ca2+ channels and Ca2+ pumps).
Our current focus is the store-operated calcium entry (SOCE) mechanism, which was initially demonstrated to play a pivotal role in lymphocyte (T cell) activation, but is now recognized as a ubiquitous process fundamental to many cell types. Recent studies identified STIM1 and Orai1 as key components of the CRAC channels that mediate SOCE in T-cells. Upon the Ca2+depletion of the endoplasmic reticulum (ER) by the IP3 receptor, STIM1 senses the low concentration of Ca2+ in the ER and sends a signal to the Orai1 channel, which forms a pore in the plasma membrane, leading to the Ca2+ influx. We employ structural methodologies to elucidate the molecular basis of this Ca2+ signalling process in order to understand (i) how the ER Ca2+depletion is trigged by IP3 and its receptor IP3R, (ii) how STIM1 functions as an ER Ca2+ sensor, (iii) how STIM1 communicates with the plasma-membrane Orai1 channel to activate the CRAC function, and (iv) how the SCID mutation may lead to disruption of the normal T cell function. We hope to understand the specific roles of Orai1 and STIM1 in cancer cells and how we may be able to manipulate their deregulated functions in cancer cells.
Acknowledgements
Research in the Ikura laboratory has been supported by public and non-profit granting agencies including Canadian Institutes of Health Research (CIHR), Canadian Cancer Society Research Institute (CCSRI), Heart and Stroke Foundation of Ontario (HSFO), Canadian Foundation of Innovation (CFI), and Princess Margaret Cancer Foundation.
For further information about our research, visit our laboratory website.
For information on “Cellular Calcium Information Server.”
For information about our NMR facility.
Recent Publications
- Smith MJ, Ottoni E, Ishiyama N,GoudreaultM, Haman A, Meyer A, Tucholska A, Gasmi-SeabrookG, Menezes S, Laister RC, MindenMD, Marschalek R, Gingras A-C, HoangT, IkuraM Evolution of AF6-RAS Association and its Implication in Mixed-Lineage Leukemia. (2017) Nature Comm. 23; 8(1): 1099.
- Spencer-Smith R, Koide A, Zhou Y, Eguchi R, Sha F, Gajwani P, Santana D, Gupta A, Jacobs M, Herrero-Garcia E, Cobbert J, Lavoieh H, Smith M, Rajakulendran T, Dowdell E, Okur MN, Dementieva I, Sicheri F, Therrien M, Hancock JF, Ikura M, Koide S, O’Bryan JP. Inhibition of Ras function through targeting an allosteric regulatory site. (2017) Nature Chem Biol 13(1); 62-68.
- Cholesterol modulates Orai1 channel function. Derler I, Jardin I, Stathopulos PB, Muik M, Fahrner M, Zayats V, Pandey SK, Poteser M, Lackner B, Absolonova M, Schindl R, Groschner K, Ettrich R, Ikura M, Romanin C. (2016) Sci Signal. 9(412):ra10
- Oncogenic and RASopathy-associated K-RAS mutations relieve membrane-dependent occlusion of the effector-binding site. Mazhab-Jafari MT, Marshall CB, Smith MJ, Gasmi-Seabrook GM, Stathopulos PB, Inagaki F, Kay LE, Neel BG, Ikura M. (2015) Proc Natl Acad Sci U S A. 112(21):6625-30.
- Cullis J, Meiri D, Sandi MJ, Radulovich N, Kent OA, Medrano M, Mokady D, Normand J, Larose J, Marcotte R, Marshall CB, Ikura M, Ketela T, Moffat J, Neel BG, Gingras AC, Tsao MS, Rottapel R The RhoGEF GEF-H1 Is Required for Oncogenic RAS Signaling via KSR-1. (2014) Cancer Cell. 25(2):181-95.
- Stathopulos PB, Schindl R, Fahrner M, Zheng L, Gasmi-Seabrook GM, Muik M, Romanin C, Ikura M. STIM1/Orai1 coiled-coil interplay in the regulation of store-operated calcium entry. (2013) Nature Commun. 4:2963.
- Findlay GM, Smith MJ, Lanner F, Hsiung MS, Gish GD, Kaneko T, Huang H, Bagshaw RD, Ketela T, Moffat J, Ikura M, Li S, Sidhu S, Rossant J, Pawson T. The binding properties of the RasGEF Sos1/Grb2 complex define timing and selectivity in embryonic stem cell lineage commitment. (2013) Cell 152(5):1008-20.
- Yang Z, Xue B, Umitsu M, Ikura M, Muthuswamy SK, Neel BG. The signaling adaptor GAB1 regulates cell polarity by acting as a PAR protein scaffold. (2012) Mol Cell 47(3):469-83.
- Wang F, Marshall CB, Yamamoto K, Li GY, Gasmi-Seabrook GM, Okada H, Mak TW, Ikura M. Structures of KIX domain of CBP in complex with two FOXO3a transactivation domains reveal promiscuity and plasticity in coactivator recruitment. (2012) Proc Natl Acad Sci U S A. 109(16):6078-83.
- Meiri D, Marshall CB, Greeve MA, Kim B, Balan M, Suarez F, Bakal C, Wu C, Larose J, Fine N, Ikura M, Rottapel R. Mechanistic insight into the microtubule and actin cytoskeleton coupling through dynein-dependent RhoGEF inhibition. (2012) Mol Cell 45(5):642-55.
- Seo MD, Velamakanni S, Ishiyama N, Stathopulos PB, Rossi AM, Khan SA, Dale P, Li C, Ames JB, Ikura M, Taylor CW. Structural and functional conservation of key domains in InsP3 and ryanodine receptors. (2012) Nature 483(7387):108-12.
- Muik M, Fahrner M, Schindl R, Stathopulos P, Frischauf I, Derler I, Plenk P, Lackner B, Groschner K, Ikura M, Romanin C. STIM1 couples to ORAI1 via an intramolecular transition into an extended conformation. (2011) EMBO J. 30(9):1678-89.
- Zheng L, Stathopulos PB, Schindl R, Li GY, Romanin C, Ikura M. Auto-inhibitory role of the EF-SAM domain of STIM proteins in store-operated calcium entry. (2011) Proc Natl Acad Sci USA. 108(4): 1337-42.
- Ishiyama N, Lee SH, Liu S, Li GY, Smith MJ, Reichardt LF, Ikura M. Dynamic and static interactions between p120 catenin and E-cadherin regulate the stability of cell-cell adhesion.(2010) Cell 141(1):117-28.
- Smith MJ, Hardy WR, Li GY, Goudreault M, Hersch S, Metalnikov P, Starostine A, Pawson T, Ikura M. The PTB domain of ShcA couples receptor activation to the cytoskeletal regulator IQGAP1. (2010) EMBO J. 29(5):884-96.
- Li GY, McCulloch RD, Fenton AL, Cheung M, Meng L, Ikura M, Koch CA. Structure and identification of ADP-ribose recognition motifs of APLF and role in the DNA damage response.(2010) Proc Natl Acad Sci U S A. 107(20):9129-34.
- Amador FJ, Liu S, Ishiyama N, Plevin MJ, Wilson A, MacLennan DH, Ikura M. Crystal structure of type I ryanodine receptor amino-terminal beta-trefoil domain reveals a disease-associated mutation "hot spot" loop. (2009) Proc Natl Acad Sci U S A. 106(27):11040-4.
- Marshall CB, Ho J, Buerger C, Plevin MJ, Li GY, Li Z, Ikura M, Stambolic V. Characterization of the intrinsic and TSC2-GAP-regulated GTPase activity of Rheb by real-time NMR. (2009) Science Signal. 2(55):ra3, 1-11.
- Stathopulos PB, Zheng L, Li GY, Plevin MJ, Ikura M. Structural and mechanistic insights into STIM1-mediated initiation of store-operated calcium entry. (2008) Cell, 135(1): 110-22.
- Hayashi I, Plevin MJ, Ikura M. CLIP170 autoinhibition mimics intermolecular interactions with p150Glued or EB1 (2007) Nat Struct Mol Biol .14(10):980-1.
- Plevin, M.J., Zhang J., Guo C., Roeder R.G., Ikura M. The acute myeloid leukemia fusion protein AML1-ETO targets E proteins via a paired amphipathic helix-like TBP-associated factor homology domain (2006) Proc Natl Acad Sci USA. 103(27):10242-7.
- Ikura, M. Ames, J.B. Genetic polymorphism and protein conformational plasticity in the calmodulin superfamily: two ways to promote multifunctionality (2006) Proc Natl Acad Sci USA. 103(5):1159-64.
- Hayashi, I., Wilde A., Mal T. K., Ikura M. Structural basis for the activation of microtubule assembly by the EB1 and p150Glued complex (2005) Mol. Cell (19)4: 449-460.
- Bosanac I, Yamazaki H, Matsu-Ura T, Michikawa T, Mikoshiba K, Ikura M. Crystal structure of the ligand binding suppressor domain of type 1 inositol 1,4,5-trisphosphate receptor. (2005) Mol Cell, Jan. 21;17(2): 193-203.
Graduate Students
Ningdi Feng Liu